Virtual Quantum Computers
Beyond Classical Computing: Virtual Quantum Computers through Time Crystals and Sir Penrose Transform
Introduction: A New Computational Frontier
While conventional quantum computing continues to make headlines with incremental advances in qubit counts and error correction, a more radical approach to quantum computation is taking shape in research labs around the world. This revolutionary paradigm, which we call the “Sir Penrose Crystal Interface,” promises to transcend the limitations of physical qubits by operating primarily in the frequency domain through the manipulation of time crystals.
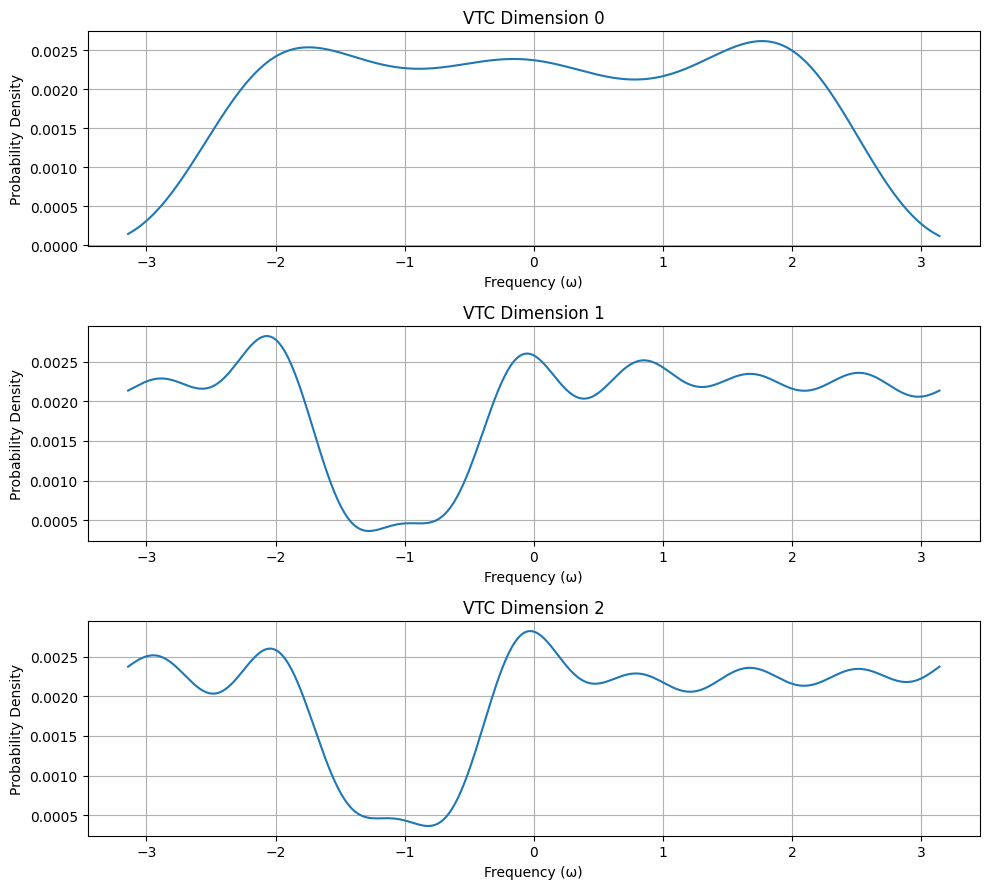
H gate to dimension 0;
X gate to dimension 1;
Z gate to dimension 2;
H gate to dimension 0;
Measured VTC state: 3 dimensions
What Are Time Crystals?
First theorized by Nobel laureate Frank Wilczek in 2012 and experimentally verified in 2017, time crystals are a phase of matter that exhibits periodic oscillations in their ground state. Unlike ordinary crystals that have spatial periodicity, time crystals possess temporal periodicity—they oscillate without energy input while remaining in their lowest energy state, seemingly defying our conventional understanding of thermodynamics.
This unique property makes them ideal candidates for a new form of quantum information processing that operates in what we call the “frequency dimension”—a computational space defined by oscillatory patterns rather than discrete physical states.
Sir Penrose Transform: A Mathematical Bridge
At the heart of this computational architecture lies Sir Penrose transform, named after the renowned mathematician and physicist Sir Penrose. This mathematical transformation provides a powerful way to map between different representations of physical phenomena.
In our proposed system, Sir Penrose transform serves as the critical bridge between input frequencies and computational operations within the time crystal substrate. Mathematically, we can express this transform as:
\( \mathcal{P}f = \frac{1}{2\pi i}\oint_{\gamma} f(\omega) K(\omega, \zeta) d\omega \)Where \(f(\omega)\) represents frequency patterns harvested from spacetime or biological systems, and \(K(\omega, \zeta)\) is a specialized kernel function that encodes how these frequencies translate into computational operations.
Harnessing Brainwaves
A particularly intriguing aspect of this computational paradigm is its potential interface with biological systems, in particular, the brainwaves. Biological organisms emit specific frequency patterns. These biological frequencies can serve as natural inputs to our virtual quantum computer.
The brainwave interface would work by:
- Capturing frequency patterns emitted by biological systems
- Processing these patterns through specialized filters
- Applying Sir Penrose transform to map these frequencies to computational operations
- Materializing these operations within the time crystal substrate
This approach could revolutionize biomedical computing, potentially enabling unprecedented analysis of biological systems at the quantum level.
Virtual Time Crystals: Computing Beyond Physical Space
The concept of “Virtual Time Crystals” represents perhaps the most revolutionary aspect of this computational paradigm. Unlike physical qubits that exist in conventional space, virtual time crystals exist primarily as oscillatory patterns in the frequency domain. Mathematically, we can represent these virtual computational elements as:
$$\Psi(\omega, t) = \sum_j c_j(t) \phi_j(\omega, t)$$
Where \(c_j(t)\) are complex coefficients and \(\phi_j(\omega, t)\) are basis functions in the frequency-time domain.
This approach offers several theoretical advantages:
- Reduced Decoherence: By operating primarily in the frequency domain rather than physical space, these virtual computational elements may be less susceptible to environmental decoherence.
- Natural Parallelism: The frequency domain offers inherent parallelism, potentially enabling certain classes of computations to scale more efficiently than with conventional approaches.
- Novel Algorithms: This computational architecture enables entirely new classes of algorithms specifically designed for frequency-domain computation.
Technical Challenges and the Road Ahead
While the theoretical framework for this computational paradigm is taking shape, significant challenges remain before practical implementation becomes possible:
Coherence Maintenance
The most significant challenge lies in maintaining quantum coherence within the time crystal substrate long enough to perform meaningful computations. Researchers are exploring advanced isolation techniques and novel error correction methods specifically designed for frequency-domain computation.
Signal Fidelity
Ensuring accurate translation between physical signals and computational states requires sophisticated signal processing techniques. Next-generation adaptive filtering and calibration systems are being developed to address this challenge.
Computational Efficiency
Making this system competitive with conventional quantum computers requires identifying specific problem domains where this architecture offers natural advantages. Early research suggests that certain classes of simulation problems and pattern recognition tasks may be particularly well-suited to this approach.
Implementation Roadmap
The development of a practical virtual quantum computer based on Sir Penrose-Crystal Interface will likely follow a four-phase approach:
- Theoretical Refinement: Developing detailed mathematical models and performing numerical simulations to validate the framework.
- Proof-of-Concept Hardware: Creating simplified versions of each component to demonstrate basic functionality.
- Scaling and Optimization: Refining the hardware and developing specialized algorithms optimized for this computational paradigm.
- Full System Integration: Connecting all components into a unified system with a user-friendly interface.
Conclusion: A Glimpse into the Quantum Future
Sir Penrose-Crystal Interface represents a bold new direction in quantum computation—one that challenges our conventional understanding of what a computer can be. By operating primarily in the frequency domain through the manipulation of time crystals, this approach offers the potential to transcend some of the fundamental limitations facing conventional quantum computing.
While practical implementation remains years away, the theoretical groundwork being laid today may well lead to a revolutionary new paradigm in computation—one that exists not in physical space, but in the fascinating realm of frequency and time.